Advanced manufacturing for multifunctional materials
We developed several advanced manufacturing techniques to fabricate multifunctional materials with controllable morphology and properties. Those materials include ceramics, perovskite, carbon nanotubes, graphene, conducting polymer, etc. It is noted that there are still many challenges of making full use of those materials as well as optimizing the synergistic effects for their composites. We employed advanced technologies such as additive manufacturing (3D printing), chemical vapor deposition (CVD), physical vapor deposition (PVD), atomic layer deposition (ALD), etc., to tailor the structure, shape, size, composition, and properties of those composite materials. We then employed those advanced materials for various applications including energy storage, wearable energy conversion, solar cells, and biomedical devices.
- Multifunctional materials with controlled properties based on Additive manufacturing
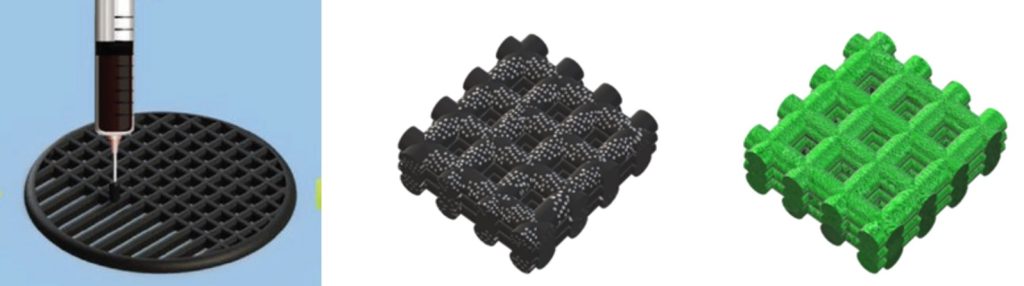
- 0D to 3D materials with CVD techniques
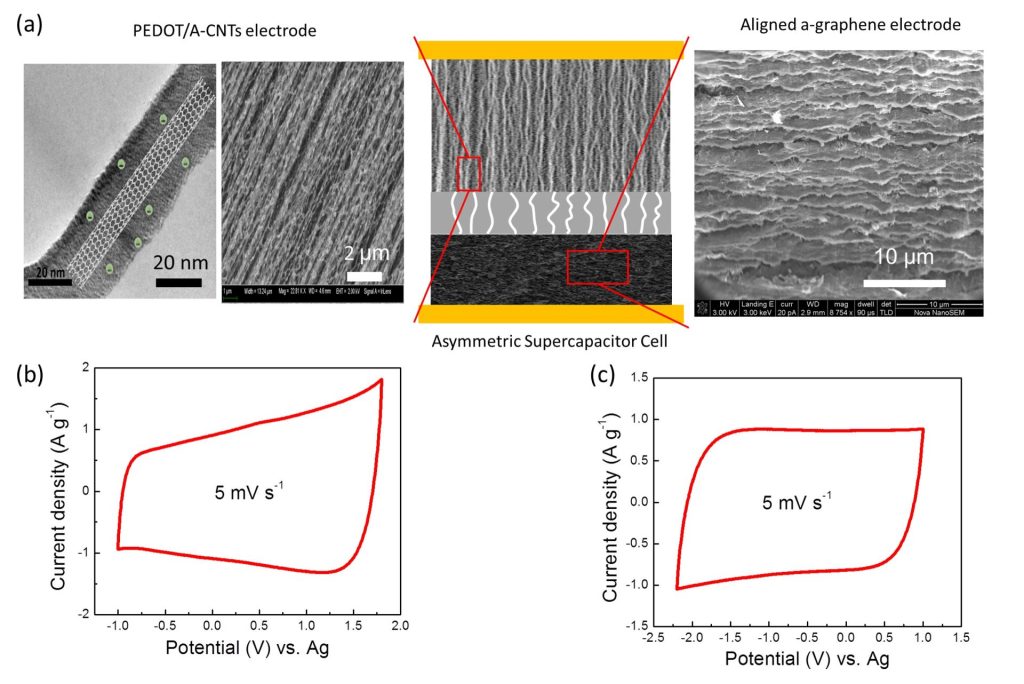
- Conducting polymers for energy storage based on oxidative CVD
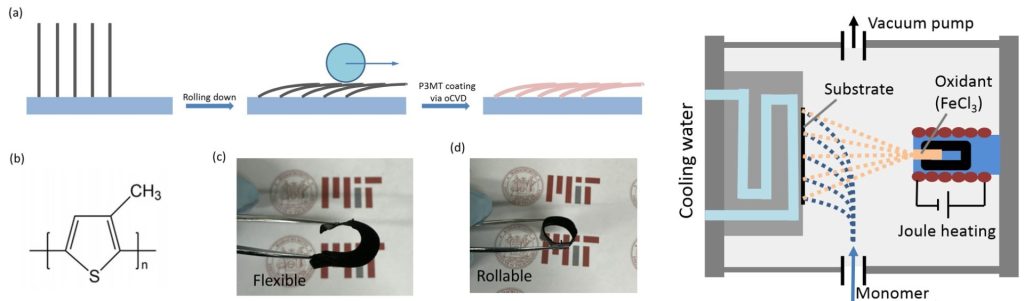
- Porous Cu foam with highly lithiophilic property

High-performance Lithium metal batteries via engineering the interface
Lithium (Li) metal, with the 10 times higher theoretical capacity than the commercial graphite anode, has been considered as one of the most promising anode materials for the next generation of energy storage devices. However, the Li metal anode has issues of dendrite growth and low Coulombic efficiency, limiting its practical applications. We employed several strategies to engineering the interface between Li metal electrode and the electrolyte with both high mechanical properties and electric properties, leading to the stabilized Li metal electrode and high performance of the battery cells.
- Flower-shaped lithium nitride as a protective layer via in-situ method for stable lithium metal anodes
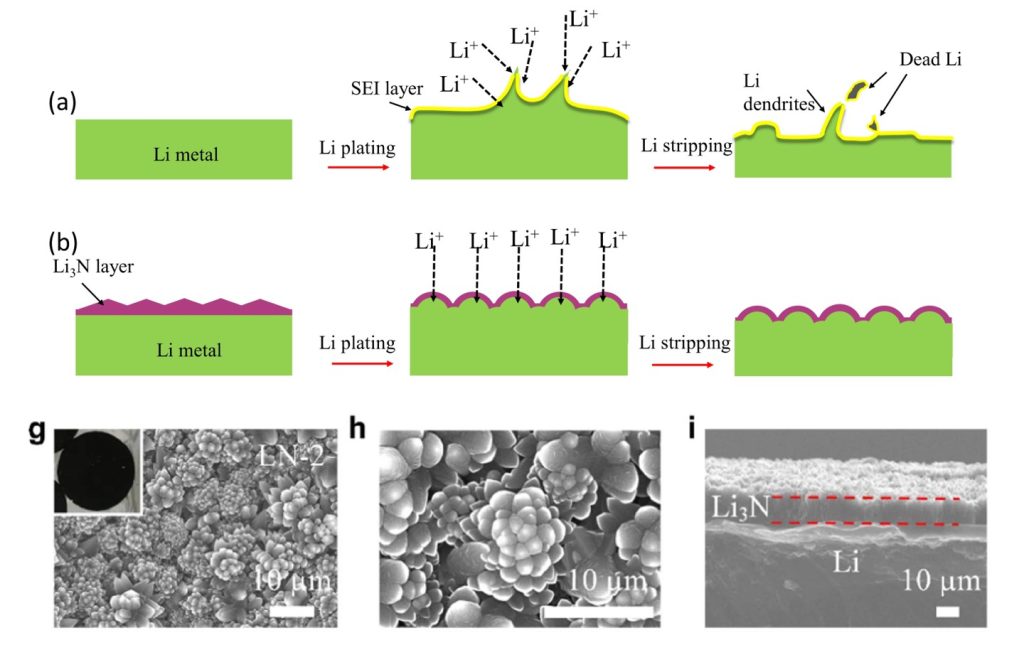
- Ultrathin Bilayer of Graphite/SiO2 as Solid Interface for Reviving Li Metal Anode
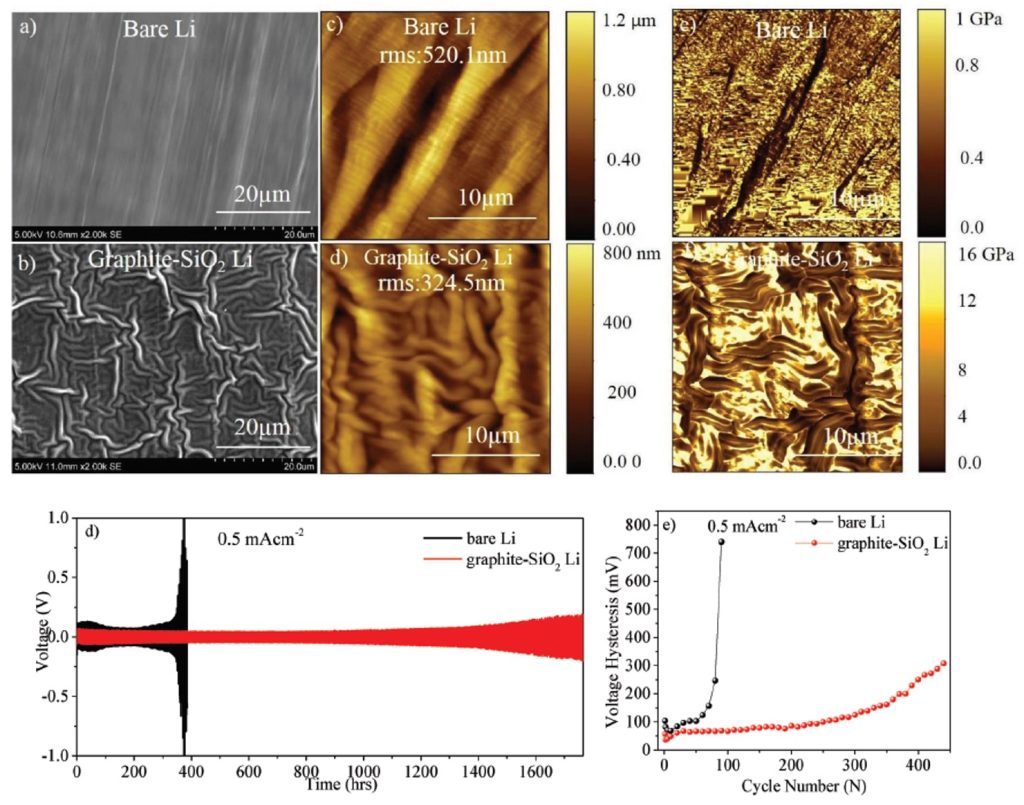
Energy storage beyond lithium ion
While we are investigating the ultimate potential of Li ion battery, the development of an alternative battery system is essential to mitigate supply chain risk of Li. Sodium (Na) and Zinc (Zn) batteries have shown their great potential, especially for large-scale energy storage applications. We are performing both fundamental and applied research on the next-generation systems by integrating the studies of electrochemistry, materials science, and mechanical stability.
- High pseudocapacitance sodium anode by an in-Situ nanocrystallization strategy
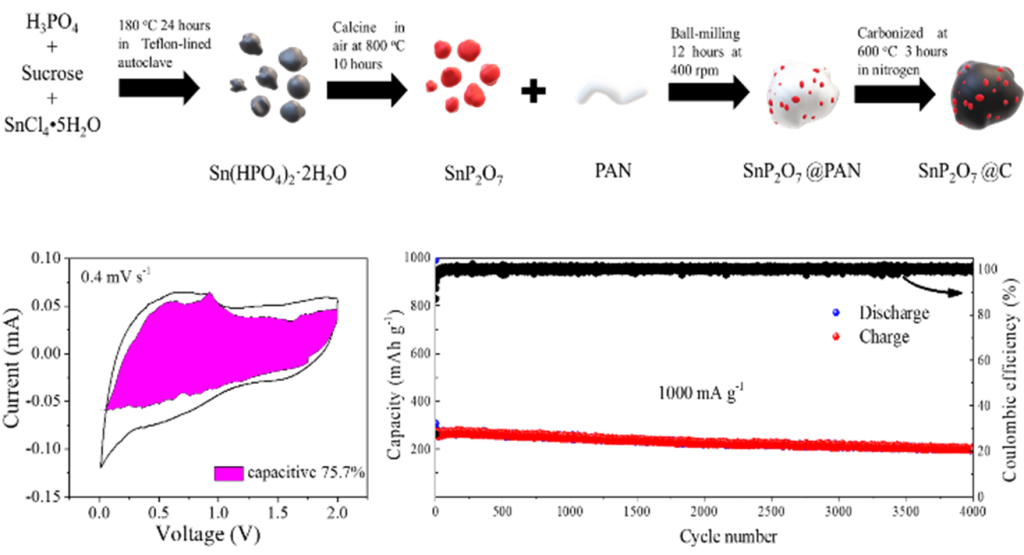
- Electrolyte additive for ultrastable aqueous Zn metal batteries
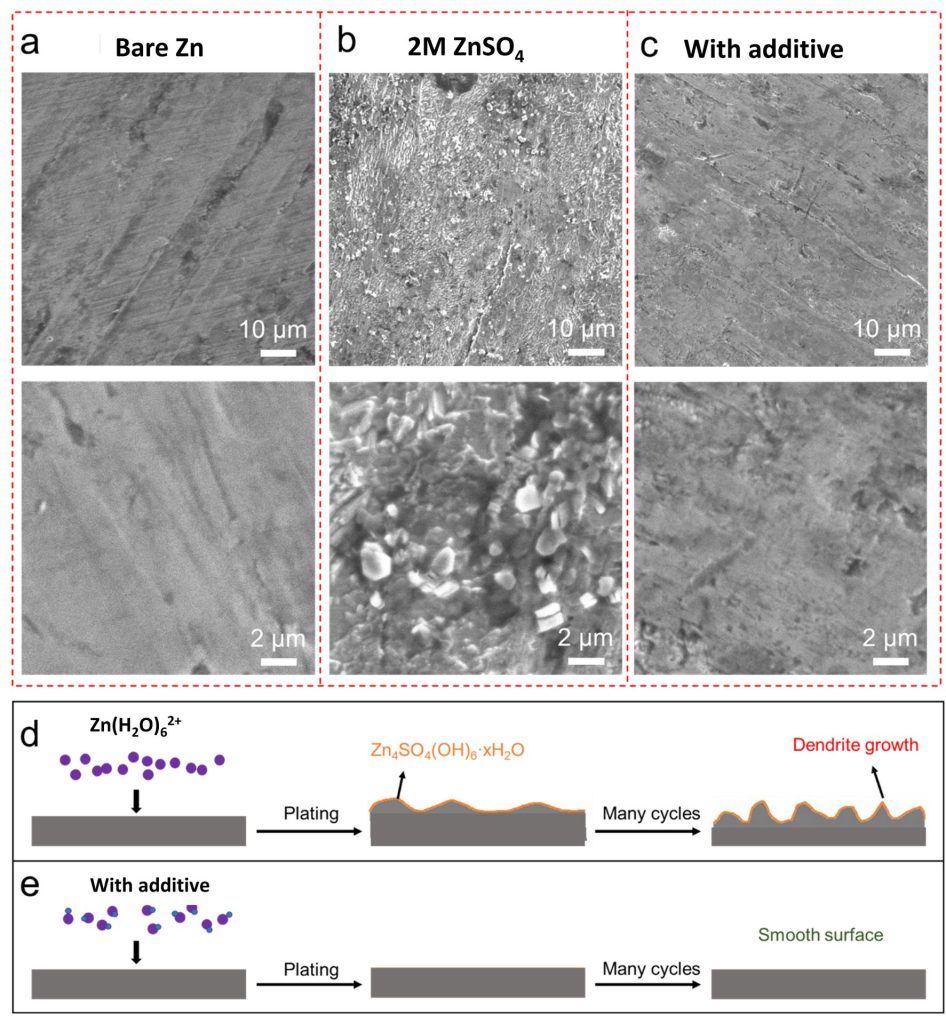
Energy harvesting devices based on heterostructure
Energy harvesting is the process in which energy is captured from one or more external energy sources and converted into electricity. The power and energy densities of all current energy harvesting devices are still very low. Moreover, although low frequency mechanical energy sources are ubiquitous, such as human motions and winds with the typical frequency values less than 1 Hz, those energy harvesting devices usually work with high efficiency only at high frequencies > 10 Hz. W designed and fabricated a flexible energy harvesting device which makes use of the heterostructure, which is reported as transducer for the first time, enabling the energy harvesting device at very low frequency. The high rectification of junction, high ion concentrations and high conductivity at the two electrodes lead to the peak power density of 0.39 mW cm-2, orders of magnitude higher compared with conventional piezoelectric energy harvesting devices.
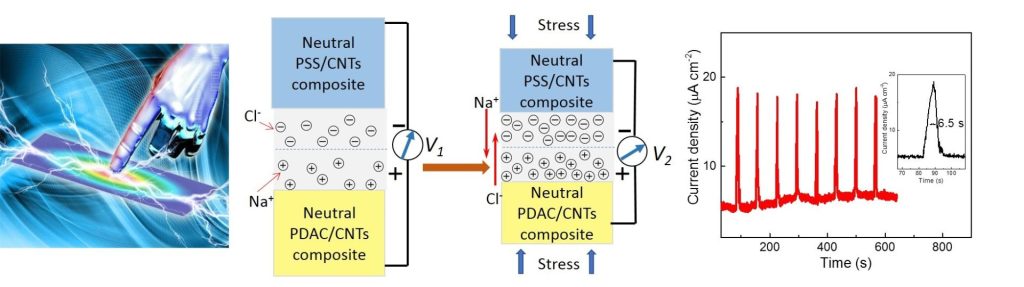