Multi-Scale Materials Modeling
Machine Learning
CT-MEAM Potential
Development of semi-empirical interatomic potential methods is important to enable large scale simulations. Nevertheless, due to the complex nature of bonding types in transition metal oxide materials, the development of reliable interatomic potentials faces significant challenges. To overcome the limitations of conventional fixed charge potential methods, we have developed a dynamic charge potential method: Charge-Transfer Modified Embedded-Atom Method (CT-MEAN). CT-MEAM uses the dynamic charge model of charge transfer interatomic potential (CTIP). to study atomic charge transfer within metal oxides, and it also uses the strengths of the advanced MEAN potential to accurately reproduce the nature of covalent and metallic bonding. Therefore, CT-MEAM describes the short-range metallic/covalent interactions via MEAM and the long-range ionic interactions through CTIP.
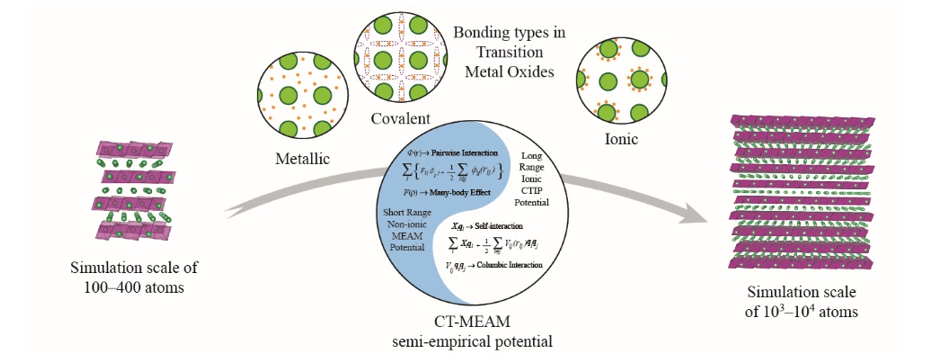
Phase Field Modeling
Kinetic Monte Carlo
Renewable Energy Materials
Li-ion Battery
The wide application of Li ion battery (LIB) on the fields of smart grid, portable electronic device, and especially, automotive, has largely promoted the renaissance of electromobility. Inside a battery system, cathode materials are the key that determines the battery capacity. Our work mainly focus on various high energy cathode materials, including Ni-rich layered oxides, Li-Mn-rich layered oxides, silicates, etc. Density Functional Theory (DFT) and Molecular Dynamics (MD) are applied to obtain fundamental understanding of different aspects of the materials, covering the materials degradation, doping effects, phase transition, interface reactions, etc.
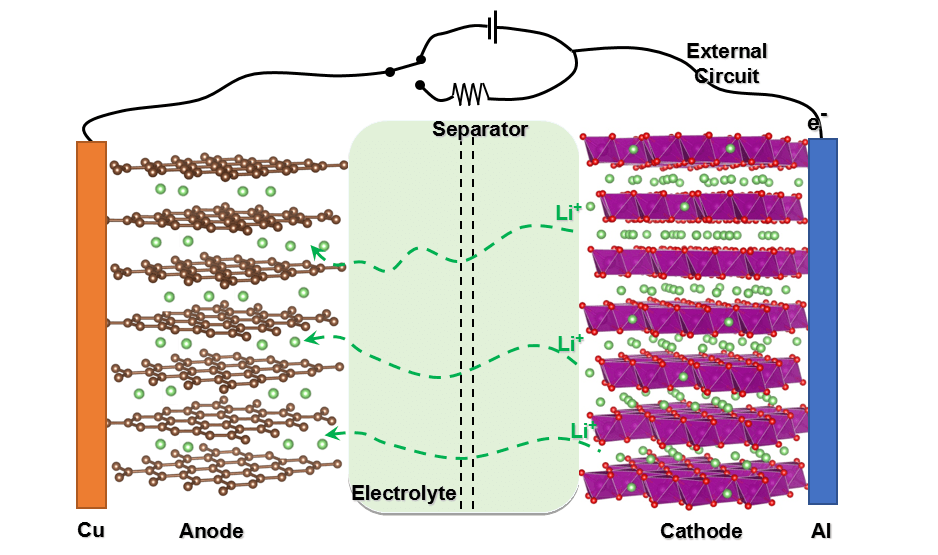
Metal-air Battery
Fuel Cell
Pollution Control
Low Dimensional Materials
2D Materials (Graphene, Transition Metal Dichalcogenides, others)
vdW Heterostructures of 2D Materials
2D/3D Interfaces
2D materials, such as graphene and TMDs, have been focused on because of their intriguing physics. It is obvious that 2D-material-based devices have been ubiquitous 2S/3D interfaces: electrical contact, channels on substrate, etc. 2D/3D interfaces show peculiar properties which have not been seen in 3D/3D interfaces. For example, as to 2D/3D top contact, van der Waals interaction has a important role; on the other hand, as to 2D/3D edge contact, chemisorption interfaces can form and lower to contact resistance. These can be predicted and interpreted by electronic structure calculations. In terms of the first principles, we explore various aspects pertaining to the 2D/3D interfaces.
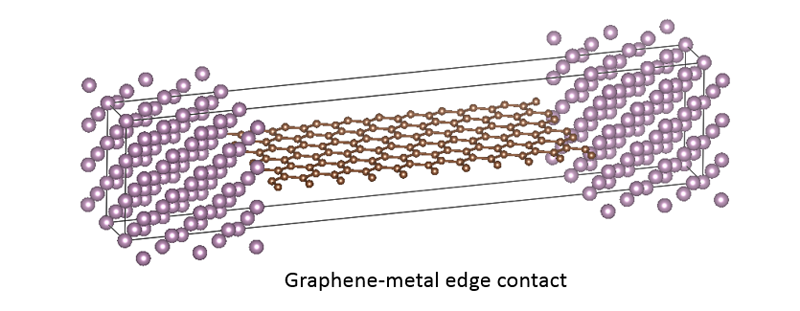